Featured Stories, MIT | April 25, 2013
‘Little Greens’ in the Age of Marine Genomics
By Genevieve Wanucha
Like seeing the world in a grain of sand, Penny Chisholm sees the inner workings of the biosphere in a tiny marine cyanobacteria. Now, a genomic revolution in biological oceanography is revealing how explosive encounters between microorganisms create ocean diversity.
In 2003, Prochlorococcus became one of the first marine microbes to have its entire set of genes sequenced. It’s an impressively small genome for the most abundant photosynthetic organism on the planet, for the source of the oxygen filling every fifth breath you take. This genus of cyanobacteria is a tiny master of ocean life, able to survive even at very low sunlight intensities and across a range of nutrient levels and temperatures. Biological oceanographer Penny Chisholm, whose team discovered ‘little greens’ in the Sargasso Sea in 1985, has devoted her entire career to understanding what regulates this microbe’s dominance in the ocean. That initial sequencing ushered Chisholm into the era of genomics, transforming her lab’s approach to their model microbe.
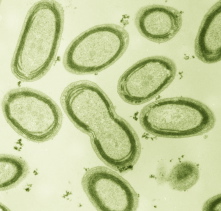
The Chisholm lab soon sequenced other individual Prochlorococcus genomes from their own collection of cultured strains. The results were revelatory. While individual Prochlorococcus genomes share a core of about 1,200 genes, hundreds of other genes vary wildly from strain to strain. In fact, to this day, each sequencing of a strain uncovers about 200 brand new genes, some never before seen at all, most of unknown function. “We slowly learned there’s this incredible genetic diversity in oceans,” says Chisholm, “which is contributing to Prochlorococcus’ resilience and abundance.”
An Ocean’s Genome
At the same time as the Prochlorococcus genome sequencing, ocean genomics was taking its greatest stride forward as entrepreneur and biologist Craig Venter launched the Global Ocean Sampling Expedition, a large-scale cruise expedition to collect samples of microbes in seawater for genetic sequencing. Fortuitously for the Chisholm Lab, Venter’s cruise traveled the warm tropical waters that Prochlorococcus inhabits, making it likely that plenty of its unique genes would be cataloged into a rapidly growing ‘metagenomic’ database, essentially a library of genes from millions of different unspecified organisms.
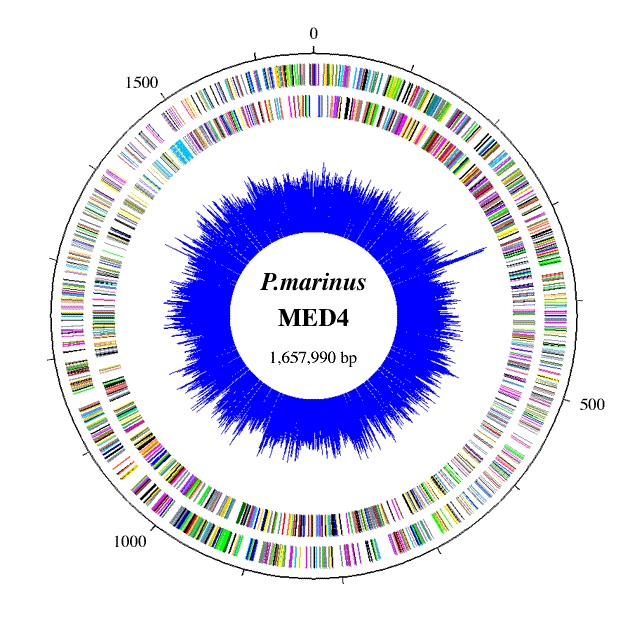
Armed with the fledgling set of metagenomic data, the Chisholm Lab started to use their individually sequenced genomes as references to locate Prochlorococcus genes in the “gemisch” of genes in the database. “Metagenomics is really what changed the way we do things,” says Chisholm. Suddenly, they were able to compare the gene pools of Prochlorococcus living in different ocean regions.
One of the lab’s first genomic era insights was that the contents of a Prochlorococcus genome depend on the environmental pressures in its habitat. For example, Prochlorococcus living in the Atlantic Ocean have many more genes for acquiring the essential nutrient phosphorous than do cells from the Pacific Ocean, which has a higher concentration of phosphorus. They concluded that Atlantic Ocean ecotypes have evolved a sensing and regulatory system that boosts the ability of microbes to find phosphorus in areas low in the nutrient. But this finding just scratched the surface of what genomics would reveal.
Close Encounters of the Viral Kind
A current focus of the lab is the mysterious interaction of viruses and Prochlorococcus in the ocean. Certain viruses contain Prochlorococcus metabolism genes, apparent vestiges of past gene-swapping encounters. Chisholm and her researchers were fascinated to find that a virus can use these genes to co-opt the host cell’s nutrient uptake machinery.
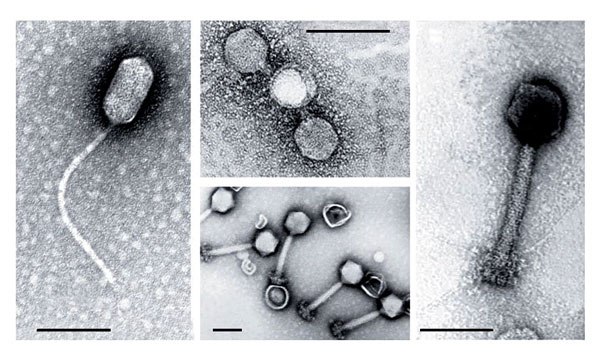
In cell culture, the Chisholm lab has demonstrated that phosphate acquisition genes in the viral genome only turn on when their host cell is exposed to environments low in the nutrient. Evidently, the virus responds to phosphate limitation and uses its own gene to pull more phosphate into the cell. This viral exploitation both satisfies the host’s need for scarce nutrients and supplies the virus with enough phosphorus to replicate its DNA, explode its host, and release progeny virus back into the ocean to continue the cycle again. In other words, the host cell and its infecting viruses have co-evolved in response to the selection pressures in their environment.
Biologist Libusha Kelly, postdoctoral associate in the Chisholm lab, didn’t have much experience in microbial ecology before joining the lab, but she had plenty with genes. “Penny took a chance on a human geneticist,” she says, itself a sign of genomics’ growing importance to biological oceanography. Kelly is now looking deep into viral genomes to understand how much viruses are affecting host metabolism. Recently, she identified sequences from Prochlorococcus-infecting viruses from high and low phosphate regions of ocean. Using both sequenced genomes from cultured viruses and metagenomic data sets, she found that in low phosphate oceans, viruses are more likely to carry a particular phosphate acquisition gene. “This suggests,” Kelly says, “that the viruses are very attuned to the environmental pressure that the host is under, and they are trying to find ways to overcome that pressure so they can successfully infect the host.” Helping the host is the same as helping themselves.
The gene trading also happens the other way around. Chisholm lab researchers now constantly spot telltale signs that viruses have been fiddling in Prochlorococcus genomes, including spots on the genome called “hypervariable islands” where it seems viruses have inserted new genes. “We think that viruses are maintaining or creating diversity that natural selection then works on,” says Chisholm. “Even though they kill the host Prochlorococcus cells, all those cells have clones, so you can afford to lose some if in the process you have a diversity creating mechanism.” As her career at MIT passes its 37-year mark, recently crowned with a National Medal of Science, Chisholm has finally glimpsed the deepest roots of Prochlorococcus’ ecological diversity, her goal since the tiny microbe became her muse.