Featured Stories, MIT Sea Grant | October 2, 2012
Saving Our Coast’s Most Valuable Marine Plant
By Genevieve Wanucha
MIT Sea Grant’s Autonomous Underwater Vehicle (AUV) Laboratory is known for its “reef explorers,” a class of remote operated vehicles used for shallow seawater missions. The newest prototype, The REX III, is now being updated. It’s been outfitted with new navigation technology. The front-facing laser sensors were reoriented to face downwards. These adjustments specifically tailor this swimming vehicle to help in government agency efforts to restore seagrass, one of the most productive marine ecosystems in our coastal waters–and the world.
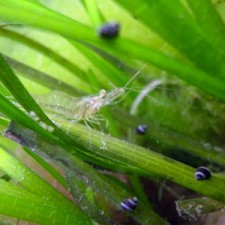
Seagrass is a flowering marine plant with long, ribbon-like blades and many services to offer. Meadows of seagrass keep sediment in place during tides, preventing coastline erosion. Our local variant, called eelgrass, provides a nursery for young lobsters, juvenile fish, and other larvae. “Eelgrass is a critical for the overall quality of coastal habitats all along the eastern coast of the US,” says Juliet Simpson, coastal aquatic ecologist with MIT Sea Grant. Intact eelgrass yields great economic value as well. A lack of these meadows, for example, means no scallops.
Because all seagrasses need to photosynthesize, they live only in shallow seawater that receives enough sunlight. As a consequence, poor water quality has, for decades, depleted eelgrass beds in Massachusetts’s bays and estuaries, most up to 70%. Ever since the Boston Harbor Project halted the direct dumping of sewage into Boston Harbor in the nineties, water quality has been improving, and attempts to coax eelgrass back to life are now worth the cost. From 2004-2007, the Department of Marine Fisheries restored over 5 acres in the harbor. Now, they need MIT Sea Grant’s engineering expertise.
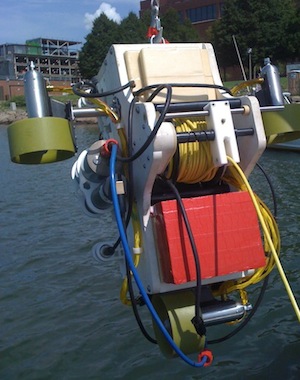
Last summer, the MIT Sea Grant’s AUV Lab brought on environmental scientist Chris McIntyre to help monitor the progress of the surviving Marine Fisheries eelgrass beds. To do this, he and his team need to survey the extent of eelgrass beds and compare the data with measures of water quality, light availability, and invasive species presence. On any given day, McIntyre and Simpson, accompanied by REX engineer Mike Soroka and lab interns, deploy the REX III from a boat. They command its navigation by remote. But the vehicle needs no human help to handle the mission’s demands; the REX can stay on a straight track in strong ocean currents and avoid obstacles, recording color video and 3D images of the eelgrass the whole time.
The AUV provides the eelgrass mission unique advantage. Present eelgrass operations use a team of human divers, which involves many safety precautions, expense, and a short time window for data collection. MIT’s underwater robot is designed as a replacement to a diver. “It doesn’t need oxygen, it doesn’t suffer from the bends, and it can run for up to 24 hours,” says Soroka. “It’s very efficient, so we’ve had a lot of luck finding what we’re looking for without a team of divers and safety officers.” In this season of the project, the team will work right up to the end of October when the water gets too cold.
MIT Sea Grant also has another eelgrass goal. “Site selection is a huge issue,” says Simpson, “and with the REX AUV, we can figure out why the restoration is not succeeding or why restorations are successful in other sites and use that information to pick new sites.” But even though researchers use several known parameters for site selection, such as light availability and sediment quality, successful replanting of eelgrass is notoriously difficult to achieve, even in adequate water quality conditions. In fact, the basics of fluid mechanics explain why the endeavor is such a challenge.
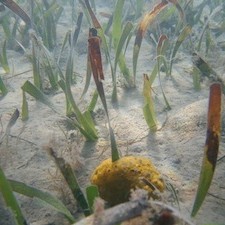
The movement of water over seagrass meadows creates drag, which removes energy from the waves flowing above, dampening their impact. The underlying sediment is not kicked up. Dense meadows therefore have less suspended sediment blocking sunlight from above, and plenty of photosynthesis can proceed. But as a seagrass patch becomes less dense, the flowing water will stir up more sediment, blocking sunlight. “So, the seagrasses will not be able to photosynthesize and will eventually die off,” says Mitul Luhar, who earned his MIT PhD in 2012 after making major headway in understanding the feedbacks between seagrass growth and hydrodynamic conditions.
Luhar comes from MIT’s Environmental Fluid Mechanics Lab, led by professor Heidi Nepf, who studies the physics of how plant geometry affects the water flow and eventually land evolution. There in the lab, Luhar soon discovered the plants. “I found there was a lot of interest in seagrasses,” he says. One reason for the growing interest is that seagrass can store twice as much carbon as the world’s forests—keeping it from releasing into our atmosphere as CO2. Luhar traveled to Mallorca, Spain, to join coastal ecologists studying the lush seagrass meadows of the Mediterranean Sea.
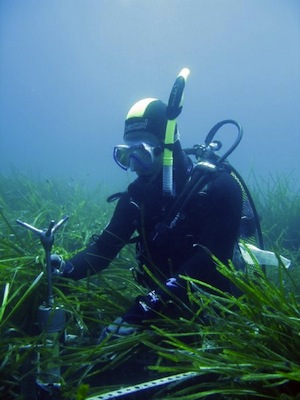
There, snorkeling over the emerald green beds of grass, he installed and operated devices to measure the speed of the waves flowing over the grasses. Luhar collected enough data to provide the ecologists with valuable information. For one, he was able to predict the limit at which a meadow’s condition switches from being healthy to one lacking enough grass to create the amount of suspended sediment that will cause die off. “That limit has implications for restorations,” explains Luhar, who is now a post-doc at California Institute of Technology, “because you must have at least a certain amount of sea grass already there for it to take hold. If you don’t have that minimum amount, there won’t be enough sunlight, and so any restoration effort will not be very useful because all the seagrass will just disappear again.” His study was published this summer in Marine Ecology Progress Series.
Luhar’s theoretical model highlights an environmental challenge. Considering that water flow over patchy seagrass beds makes it difficult for seedlings to take hold in sediment, how will researchers re-create the hydrodynamic conditions for successful regrowth? There’s a long way to go to answer that one. As Heidi Nepf points out, “We don’t even know answers to questions as simple as: what is the optimum spacing between seedlings to ensure the stability of the meadow?” The eventual goal of the Nepf lab researchers is to help in site selection for restoration projects to maximize success. And if other students follow in Luhar’s steps, perhaps that goal is not as far off as it seems.
Back at the MIT Sea Grant, the AUV lab’s monitoring efforts are turning up some encouraging signs. Eelgrass is growing in places where there was none five years ago, and a long-term improvement is undeniable. “This year relative to a decade ago, we are looking good,” says Soroka. Additionally, new environmentally conscious boat moors in Manchester-by-the-Sea, which save eelgrass from the lawn-mower effect of traditional moors, is panning out. “The eelgrass definitely seems be growing back into those areas, which is positive,” says McIntyre. However, the high cost of the new moors will complicate a wide-spread switch-over. Another problem is that the Boston Harbor water quality, though showing improvements, still sometimes makes it hard to examine biological details in the eelgrass beds.
The sensor-clad robot driving over the floor of the Boston Harbor may seem a small project. And hydrological models of water-plant interaction may, at first, seem a narrow academic topic. In fact, these efforts are part of increased scientific attention to one of the world’s most valuable and threatened ecosystems, one which stores carbon many meters deep and supports communities, human and animal alike.