Featured Stories, MIT, WHOI | December 29, 2012
Basic Needs: A Report on Ocean Acidification
by Kenrick Vezina
Ocean acidification is just what it sounds like: a process by which the ocean, particularly its surface, is slowly becoming more acidic. The immense amounts of carbon dioxide (CO2) being pumped into the atmosphere not only affect the planet by acting as a greenhouse gas, but also dissolve into the surface of the oceans, and react with seawater to form carbonic acid (H2CO3). Consider carbonic acid’s chemical cousin, acetic acid (that is, vinegar) and its reaction with baking soda, a base. It is here, in the reactivity of acids and bases, where the real danger of ocean acidification—and its connection to corals—lies.
Calcifers in Crisis
The addition of CO2 reduces the concentration of carbonate (CO32-), a base, in seawater. The ocean is full of organisms known as calcifers—creatures large and small (and very small), which use carbonate and calcium ions dissolved in seawater to construct their shells and skeletons of calcium carbonate (CaCO3), a base. Most famous among the marine calcifers are the corals, which build reefs of CaCO3 that are some of the greatest hotspots of biodiversity in the ocean.
Corals are hardly alone in their dependence on the carbonate dissolved in seawater. Other calcifers include marine snails (pteropods); shellfish (clams, etc.); crustaceans such as shrimp and lobsters; starfish, sea urchins and their kin (echinoderms); even single-celled amobae called foraminifera and photosynthetic algae called coccolithophores, which encase themselves in calcium carbonate platelets. Many of these organisms serve fundamental roles in marine ecosystems, either as food for larger organisms or in the biochemical processes that shape oceanic environments and the climate.
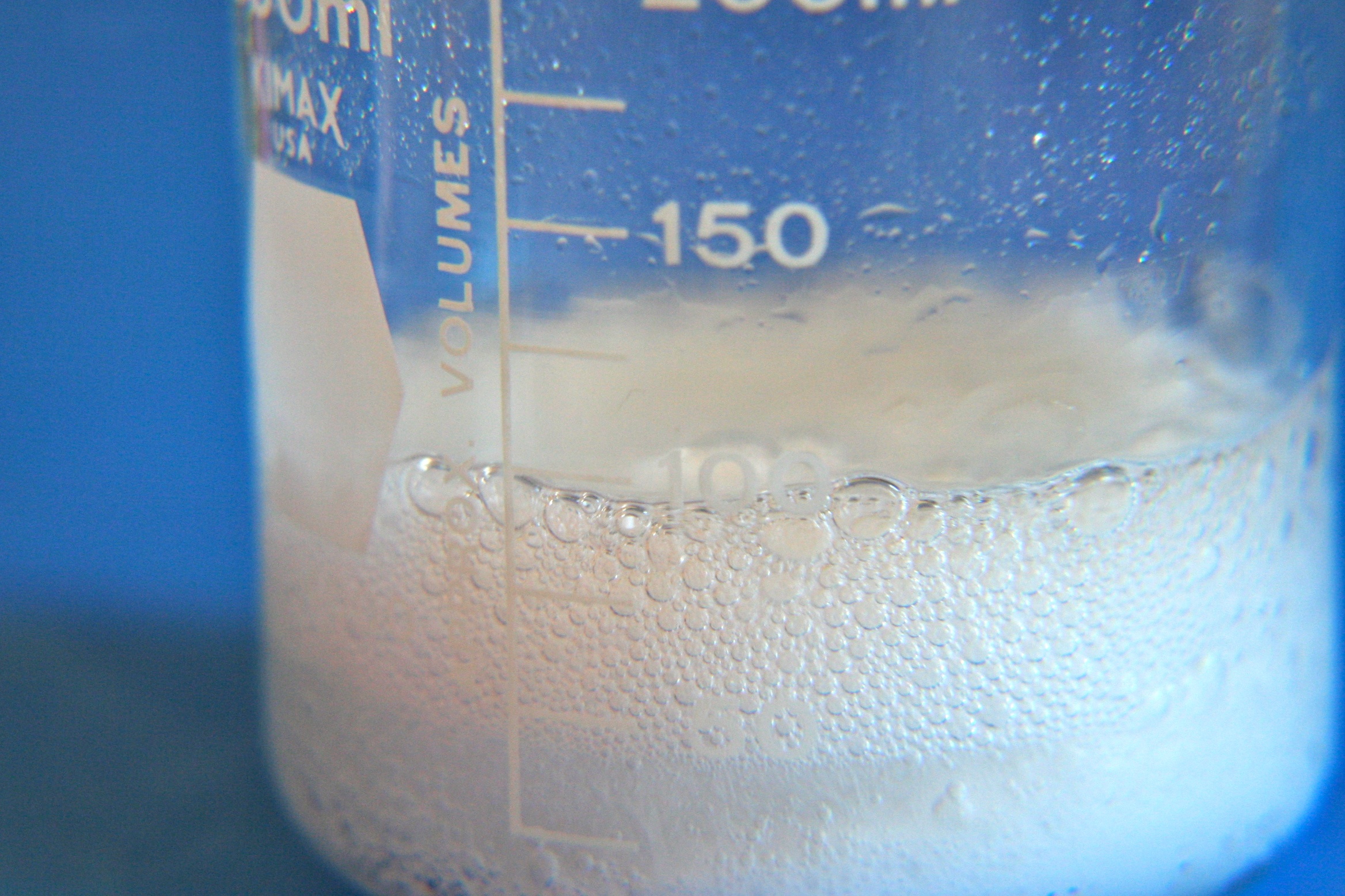
Although calcium carbonate is a base, like baking soda, and our ocean is becoming a little bit more like vinegar, this does not mean that all marine calcifers are suddenly going to find their skeletons vanishing in a flash of bubbles. What will happen is rather more subtle.As the oceans become more acidic, the concentrations of carbonate ions available in the water for calcifers to use will decrease. This will make it harder for some species to build their shells and skeletons. And, in more extreme cases, yes, their shells may begin to dissolve. Every living being has a finite amount of energy to spend on growth, maintenance (an immune system, for instance), and reproduction. In essence, acidification will make it more energy-expensive for calcifers to create their shells, stressing them and potentially leading to decreased disease resistance or reproduction.
Faster Than You Might Think
Part of what makes acidification such a pressing concern is that it has become a problem in two hundred years or so since the Industrial Revolution. There is strong evidence that the recent acceleration in the rate of ocean acidification, possibly more than any other consequence of increased carbon levels worldwide, is a direct result of human activity.
A recent study by Bärbel Hönisch, of Columbia University’s Department of Earth and Environmental Sciences, examined 300 million years of the ocean’s geological history with regards to ocean acidification and found few parallels in Earth’s history to the rate of acidification seen today. She and her team of international co-authors compared the current state of the ocean to a major acidification event that took place approximately 56 million years ago during the Paleocene–Eocene Thermal Maximum (PETM)—a period of intense warming and increased atmospheric carbon. This event bears some similarities to human induced changes, only we’re on-track to achieve similar levels of acidification in much less time than it took during the PETM. “We are doing in 200 years what took 5,000 years [in the geologic record],” she said in an interview.
What Hönisch’s study emphasizes is that it is much faster and easier to increase the ocean’s acidity than to decrease it. What we’re doing in a few hundred years may take the planet’s natural mechanisms, such as erosion of basic compounds from the land into the sea, tens of thousands of years to counteract.
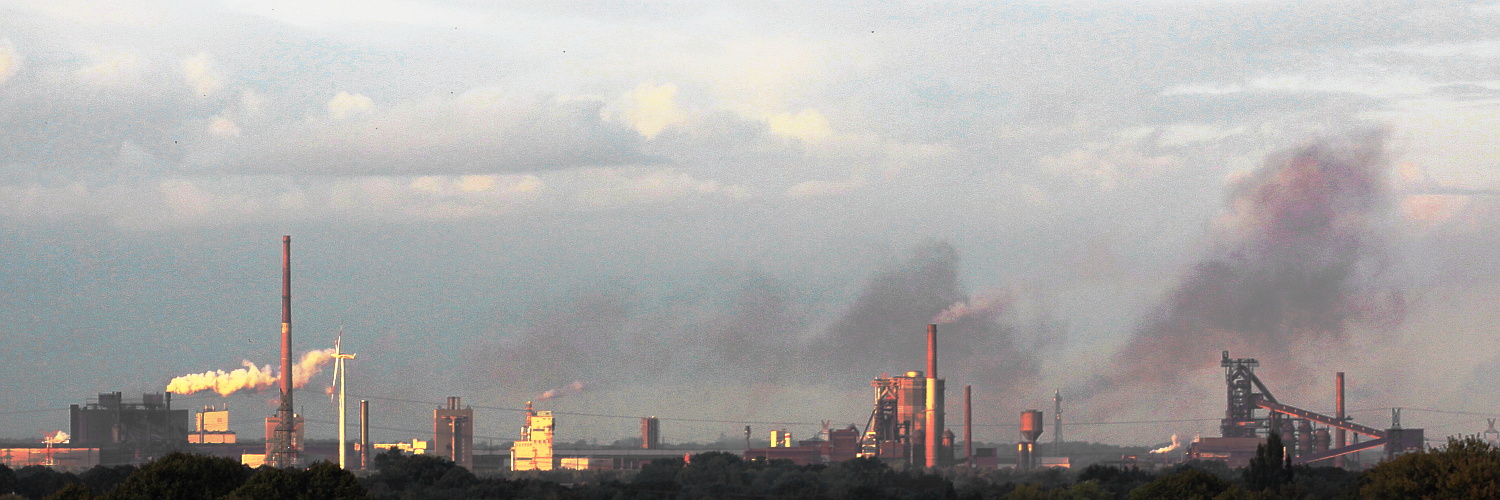
Given the long, long time it will take for the current level of acidity in the ocean to reverse via natural mechanisms, and the impracticality of direct intervention, the best bet for combating ocean acidification is prevention. There’s not much we can do to reverse the current state of things, but by cutting CO2 emissions we could slow the rate of acidification and allow the ocean’s natural mechanisms to get to work. In the interim, researchers at MIT and Woods Hole are at the forefront of trying to understand the effects of ocean acidification. Before any direct steps can be taken to prevent damage to marine ecosystems, we must first understand what that damage might be.
Anne Cohen: Studying Corals at WHOI
Anne Cohen at the Woods Hole Oceanographic Institution, is studying the effects of acidification on corals, taking into consideration the entire coral reef and its surrounding microchemistry. Cohen’s lab melds experimentation, field work, and modeling. When it comes to climate change, of which acidification is one symptom, “it’s all intertwined, there’s lots of feedbacks.” In other words, you can’t have increased CO2 without warming and acidification in tandem. In this light, her lab’s integrative approach seems a necessity.
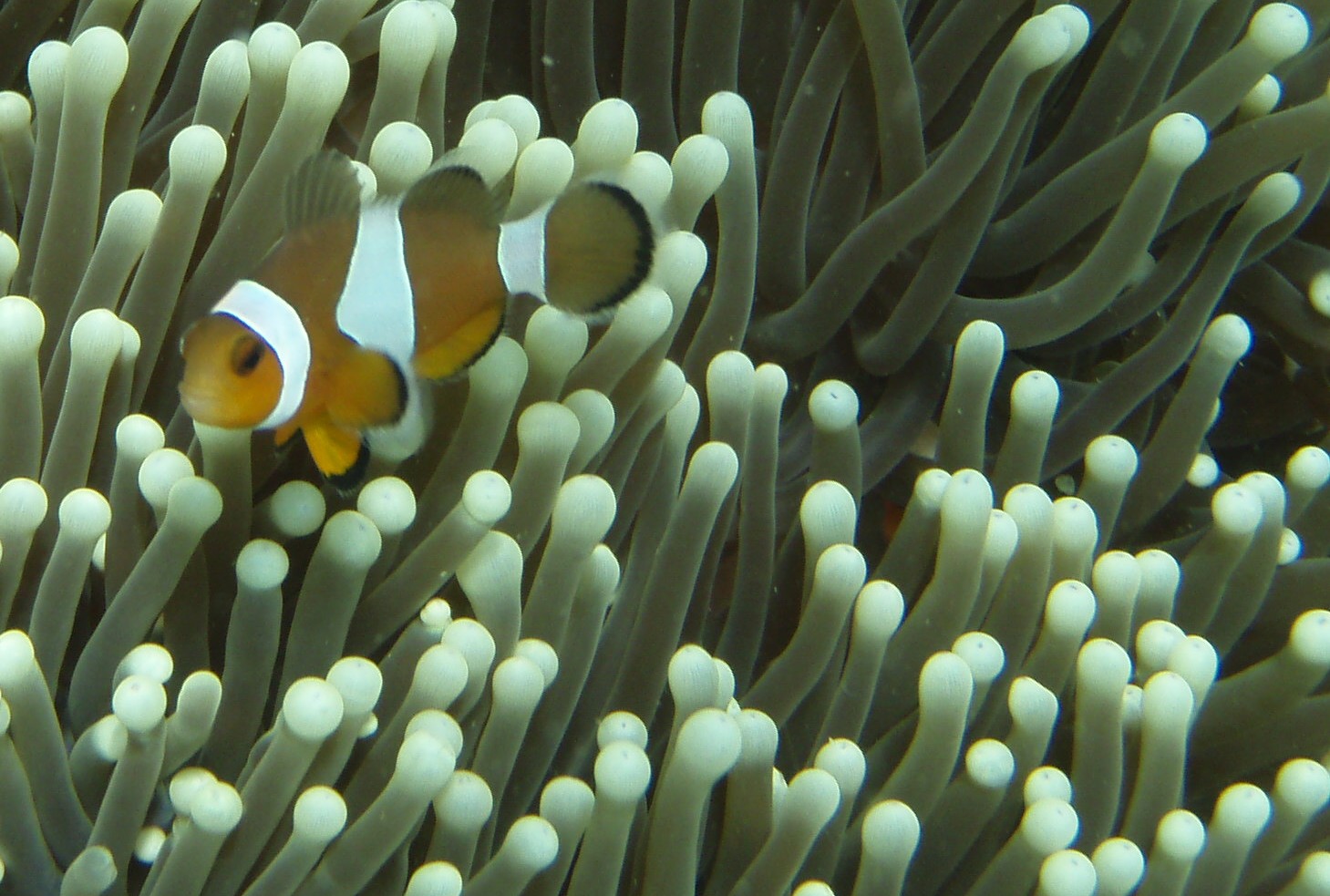
Her team exposes corals and shellfish to predicted levels carbonate saturation in the lab based on climate change models and field studies. They use this lab-work and oceanographic models to direct their field studies, which monitor how coral communities are responding to rising temperatures and increasing acidification. The field work also involves collecting samples for further lab work. And all of the data from field- and lab-work gets fed back into the models they use to make them more accurate.
Despite what current models predict for ocean acidity, Cohen was shocked to discover that “there is completely different chemistry on actual reefs.” This is due in part to the structure of reefs, she says: an outer reef with “dense, fortress-like skeletons sitting out there getting bashed by waves” creates a lagoon (inner reef) where corals and fish “have a really easy time” sheltered from the open ocean.
Janelle Thompson: Analyzing Reef Microbes at MIT
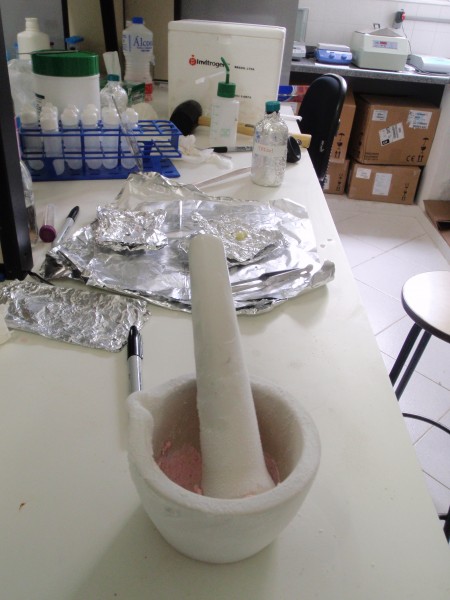
Often the conditions on inner reefs are warmer and more depleted of carbonate than those observed in open ocean in the same area. As water passes through the outer reef, it is depleted of carbonate by the fast-growing corals there. Once inside the shallower inner reef, water warms more quickly. Corals are “amazing crystal factories,” says Cohen, and the effect of their chemical wizardry on the microchemistry of their immediate surroundings seems much larger than previously assumed. This conjures many new questions, not least of which is whether or not inner reefs will do better in an acidifying ocean than outer reefs.
At MIT, Janelle Thompson is exploring another underappreciated aspect of marine life in an acidifying ocean: microbial communities. Specifically, those associated with corals. At first glance, corals may seem like a single organism, but they are in fact colonies, communal organisms. A reef-building coral contains, on average, thousands of polyps—the “animal” that envelopes the calcium carbonate skeleton. Each polyp has photosynthetic symbiotic algae that live with it, providing the polyp with food from the sun. On top of this well-known symbiosis, there is a microbial community that coexists with each coral.
An Uncertain Future
Thompson and her colleagues use genome sequencing techniques to “read” about the nature and activities of these coral-living microbes via the genes they express. With acidification a concern in the back of every marine biologist’s mind, Thompson finds herself asking questions about the potential interaction between acidification, marine microbes, and corals. “Will acid-stressed corals be more susceptible to infection? Will it change the ratio of pathogenic to non-pathogenic microbes?” Nobody is sure yet where microbes fit into an acidifying ocean. To paraphrase Thomspon: there is a robust absence of consensus.
Meanwhile, warming continues to be a concern for corals, as high temperatures often result in “bleaching”—the stress-induced expulsion of their photosynthetic symbionts and eventual death. How corals and the calcifer kin will respond to the two-pronged attack of a warming surface ocean and depleted supplies of vital carbonate remains to be seen, but the prospects are grim. “We’re still in the early stages [of this research],” says Cohen, “but I’ve not yet seen any immunity.” —Kenrick Vezina