Featured Stories | February 8, 2016
Predators in the Plankton
By Cassie Martin
This is the first article of a three-part series covering the Department of Earth, Atmospheric, and Planetary Sciences (EAPS) lectures on “Life in the Ocean.”
To kick off the New Year, EAPS invited three Woods Hole Oceanographic Institution (WHOI) scientists to share their research with the MIT community. “WHOI scientists are studying the diversity of life in the oceans. These organisms occupy every possible niche you can think of, from the surface ocean to hydrothermal vents on the sea floor,” said MIT Professor Mick Follows, who organized the event. “We want to get a flavor of what’s living in the ocean, how they’re living, and why the system is that way.”
The first speaker was Matt Johnson, a WHOI researcher who studies predator-prey interactions among the ocean’s smallest inhabitants. The term marine predator often conjures images of great white sharks or killer whales, but rarely does one think of plankton—a diverse group of microscopic organisms that are the foundation of ocean ecosystems. These tiny predators play a critical role in maintaining ocean health that rivals that of their mammalian counterparts.
Johnson is interested in how the plankton-prey microcosm affects ocean nutrient cycling, primary productivity, and species evolution. In his talk, he introduced three species that illustrate just how wonderfully weird and complex life in the ocean can be.
Chewing the Fat
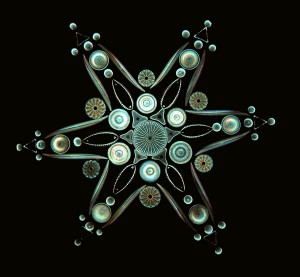
Diatoms are single-celled organisms which help drive ocean biogeochemical cycles by producing 20 percent of Earth’s atmospheric oxygen and 40 percent of the ocean’s dissolved oxygen. They also happen to be a favorite meal of marine crustaceans called copepods.
When copepods begin feasting, diatoms release oxylipin—a lipid known to disrupt copepod reproduction cycles, offspring development, and reduce copepod hatching. Using field and lab-based molecular experiments, Johnson probed how oxylipins protect diatoms by altering the cellular metabolism, growth, behavior, and mortality of predatory copepods.
He found that in response to stress from the presence of predators, diatoms produce nitric oxide. The chemical acts as a signal to other diatoms to raise their defenses in the form of oxylipin production. Johnson observed that the presence of oxylipin reduced grazing by predators. He calls chemicals such as nitric oxide “infochemicals” because they facilitate communication among species in a community of organisms.
Fewer predators means diatom blooms last much longer, which increases the amount of carbon and silicon pumped from the surface to the deep ocean. There are some trade-offs, however. While oxylipin disrupts predator reproduction, it also slows diatoms’ growth rate. Johnson is currently investigating how infochemical signaling influences fluxes of carbon and nutrients in the ocean.
Ehux in Wonderland
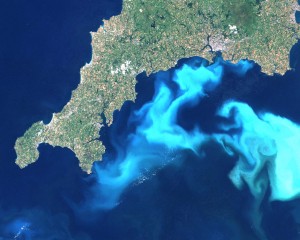
(Credit: PD-USGOV-NASA/Wikipedia)
One of the ocean’s oldest and most prolific denizens are coccolithophores—single-celled algae that have shell-like skeletons. These armored plankton, which have lived for millions of years, permeate modern oceans, fixing carbon and producing atmospheric gasses. Understanding how coccolithophores live and adapt to environmental change is increasingly important in the face of ocean warming and acidification.
Johnson focuses on a species of coccolithophore named Emiliania huxleyi, or Ehux for short. Ehux is known for its massive blooms, which can be seen from outer space. These blooms reflect light and heat back into the atmosphere, and act as a large carbon sink in the ocean carbon cycle. Johnson is interested in how interactions between Ehux’s two main foes—predators and viruses—impact its ecology and ocean ecology
Viral infection is a main cause of Ehux mortality and is well-studied by researchers. Although the Ehux virus (EhV) is one of the largest-known viruses, Ehux has a trick up its sleeve to avoid infection—a process scientists have dubbed the “Cheshire Cat Response,” after the invisible cat from Alice in Wonderland. When an Ehux population detects a virus, Ehux cells signal one another to revert to their unarmored haploid state, rendering the cells undetectable to the virus.
It’s not as easy for Ehux to escape zooplankton predators. Zooplankton don’t discriminate based on Ehux morphology or atmospheric gas production—every organism is potential food regardless of shape or size.
But Ehux is not completely defenseless. Johnson found that when armored Ehux are ingested by plankton, Ehux produces high levels dimethyl sulfide gas which halts zooplankton growth. Why this happens is still a mystery, but Johnson has a theory he’s dubbed the Tums hypothesis. Basically, once eaten, the gas and Ehux’s calcium carbonate shell react with the zooplankton’s internal pH and destroy the predator.
But how do zooplankton react to EhV-infected Ehux? In laboratory experiments, Johnson found that zooplankton graze on infected Ehux less often than on uninfected prey. “These findings open the door for a greater role for viruses in carbon sinking,” Johnson said.
Planktonstein’s Monster
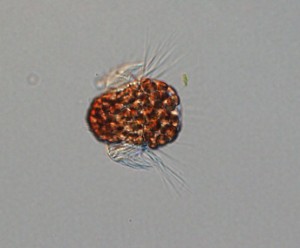
Mesodinium rubrum is Frankenstein’s sea monster. The microscopic ciliate feeds on algae but takes over use of its organelles. “Cells are a chimeric medley of prey components and its own cellular components,” Johnson said. Without the mitochondria, plastids, and nuclei of its algal prey, M. rubrum couldn’t perform photosynthesis.
M. rubrum is known as a mixotroph—it can consume prey and photosynthesize. Mixotrophy is a widespread phenomenon in marine microbial food webs, providing much needed flexibility in areas of the ocean where nutrients are scarce. However, researchers have a limited understanding of the cellular mechanisms that regulate it. Johnson studies the physiological and ecological role of mixotrophy in marine phytoplankton. “Understanding these processes is important for understanding this species’ basic ecology and primary production in their environment,” Johnson said.
Using a variety of molecular techniques, Johnson and his colleagues have learned that the gene expression of prey nuclei and protein expression patterns of other organelles change radically once consumed by M. rubrum. The stolen nuclei become dominant inside M. rubrum, suppressing most protein expression except for that involved in photosynthesis, oxidative stress reduction, and several other metabolic pathways.
But M. rubrum also exerts some control over its hostage organelles. Host genes involved in protein transport are significantly under-expressed, indicating that M. rubrum’s endomembrane system still plays a role in targeting acquired proteins and plastid complexes. In other words, while the stolen organelles dominate gene and protein expression, M. rubrum ultimately gets the final say in how the proteins and plastids are used. Other research has shown that the number of acquired plastids decrease during times of starvation.
In a rapidly warming world, ocean warming and acidification will undoubtedly affect plankton ecology. A recent study from Oceans at MIT researcher Stephanie Dutkiewicz found that increased ocean acidification over the next 85 years may fundamentally alter the ocean’s phytoplankton diversity and stratification: some species could die out while others could flourish and move toward the poles, with potential serious consequences for the food web and biogeochemical cycles.
It’s hard to say how [mixotrophy] will affect future oceans,” Johnson said. “But it’s an important process in all surface ocean microbial food webs.”